More information: Evidence for direct molecular oxygen production in CO2 photodissociation, Science 3 October 2014: Vol. 61-64.DOI: 10.1126/science.1257156. O I Ground State 1s 2 2s 2 2p 4 3 P 2 Ionization energy 109837.02 cm-1 (13.61805 eV) Ref. MG93 O II Ground State 1s 2 2s 2 2p 3 4 S° 3 / 2 Ionization energy 283270.9 cm-1 (35.1211 eV) Ref.
1 day ago MOXIE works by separating oxygen atoms from carbon dioxide molecules, which are made up of one carbon atom and two oxygen atoms. A waste product, carbon monoxide, is emitted into the Martian atmosphere. The conversion process requires high levels of heat to reach a temperature of approximately 1,470 degrees Fahrenheit (800 Celsius). Understanding Atomic Oxygen Oxygen comes in several different forms. The oxygen that we breathe is called O 2 —that is, it is comprised of two atoms of oxygen. O 3 is ozone, such as occurs in Earth's upper atmosphere, and O (one atom), is atomic oxygen. Water is made up of two hydrogen (H) atoms and one oxygen (O) atom. The formula for water is H 2 O. The hydrogen atoms have filled orbitals with two electrons.
- >News & Features
- >News Topics
- >NASA Technologies
- >Features
- Send
Feature
Text Size
But all is not lost. The painting is placed in a vacuum chamber and an invisible, powerful substance called atomic oxygen is created inside the chamber. Over the course of hours or days, slowly but surely, the grime dissolves away, and the colors begin to re-emerge. With a touch of freshly sprayed clear varnish, the painting returns to its glory.
It may seem like magic, but it is science. A scientific method developed by researchers at NASA's Glenn Research Center uses atomic oxygen to save and restore works of art that would have been irreparably damaged. It can also completely sterilize surgical implants intended for human bodies, dramatically reducing the risk of inflammation. It can improve glucose monitoring devices for diabetic patients, using a fraction of the amount of blood that was previously required for testing to manage their disease. It can texture the surfaces of polymers to invite bone cell adhesion, leading to a variety of medical advances.
And this powerful substance can be created out of thin air.
Understanding Atomic Oxygen
Oxygen comes in several different forms. The oxygen that we breathe is called O2—that is, it is comprised of two atoms of oxygen. O3 is ozone, such as occurs in Earth's upper atmosphere, and O (one atom), is atomic oxygen.
Atomic oxygen doesn't exist naturally for very long on the surface of Earth, as it is very reactive. But in space, where there is plenty of ultraviolet radiation, O2 molecules are more easily broken apart to create atomic oxygen. The atmosphere in low Earth orbit is comprised of about 96% atomic oxygen. In the early days of NASA's space shuttle missions, the presence of atomic oxygen caused problems.
'In the first few shuttle flights, materials looked frosty because they were actually being eroded and textured,' says Bruce Banks, a senior physicist with Alphaport, supporting the Space Environment and Experiments branch at Glenn. 'Atomic oxygen reacts with organic materials on spacecraft exteriors, gradually damaging them.'
Glenn Research Center was asked to investigate the damage caused to NASA spacecraft by atomic oxygen. The researchers not only invented methods to protect spacecraft from atomic oxygen; they also discovered a way to harness the potentially destructive power of atomic oxygen and use it to improve life on Earth.
Preventing Erosion in Space
Atomic Oxygen is created in a lab by separating O2 (the oxygen that naturally appears in Earth’s atmosphere) into O (one atom of oxygen—otherwise known as atomic oxygen). Photo Credit: NASAWhen spacecraft travel in low Earth orbit (where crewed vehicles and the International Space Station fly), the atomic oxygen formed from the residual atmosphere can react with spacecraft surfaces, causing damage to the vehicle. When the solar arrays were designed for the Space Station, there was a concern that the solar array blankets, which are made of polymers, would quickly erode due to atomic oxygen.
NASA developed the solution. The team at Glenn designed a thin-film coating for the solar arrays, which was immune to the reaction with atomic oxygen. Silicon dioxide, or glass, has already been oxidized so it cannot be damaged by atomic oxygen. The researchers created a coating of a clear silicon dioxide glass that is so thin, it is flexible. This protective coating adheres to the polymers of the array and protects the arrays from erosion, while not sacrificing any thermal properties. The coatings continue to successfully protect the Space Station arrays, and were also used for the arrays (supplied by the United States) for Mir.
'It has been successfully flying in space for more than a decade,' Banks says. 'It was designed to be durable.'
Harnessing the Power
Through the hundreds of tests that were part of developing the coating that was resistant to atomic oxygen, the Glenn team became experts in understanding how atomic oxygen works. The team imagined other ways that atomic oxygen might be used in a beneficial manner, rather than the destructive influence it is in space.
'We became aware of how the surface chemistry changes, how it [atomic oxygen] removes organic materials… it can remove anything organic that's a hydrocarbon, that might not be easily removed by normal chemicals,' Banks says.
The team discovered a wealth of ways atomic oxygen might be employed. They learned that it turns the surfaces of silicones into glass, which can be helpful in creating components that need to form a tight seal without sticking to each other. This treatment process is being developed for use on seals for the International Space Station. They also learned that it can restore and save damaged artwork, enhance materials used on aircraft and spacecraft, and benefit humans through a wealth of biomedical applications.
There are different ways of applying atomic oxygen to surfaces. Most frequently, a vacuum chamber is used. These chambers range from the size of a shoebox to a chamber that is 4 feet by 6 feet by 3 feet. Microwaves or radiofrequency waves are used to break the oxygen into oxygen atoms—atomic oxygen. A sample of polymer is placed in the chamber and its erosion is measured to determine the level of atomic oxygen inside the chamber.

A variety of surfaces can be treated using these methods. As research into atomic oxygen has continued, various industries have learned of the work. Partnerships, collaborations and spinoffs have begun—and in many cases, completed—in multiple commercial areas.
Helping Human Health
Atomic oxygen can be used in medical applications, such as texturing surfaces for use in glucose monitors. Photo Credit: NASAThere are many biomedical applications of atomic oxygen. Many have been investigated, and many more areas can be explored.
Atomic oxygen has been used to texture the surface of polymers that may fuse with bone. The surface of smooth polymers typically discourages adhesion with bone forming cells, but the atomic oxygen creates a surface where adhesion is enhanced. There are a variety of ways this could be beneficial to osteopathic health.
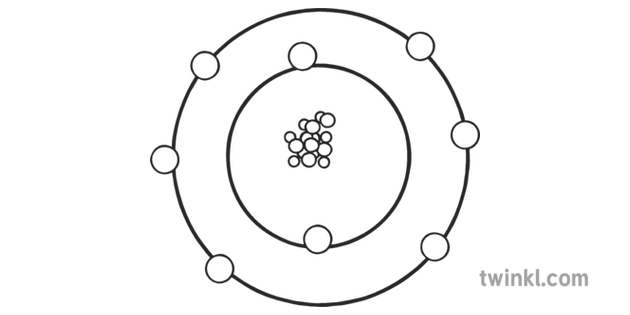
Atomic oxygen cleans the implant and removes all traces of organic materials, which greatly diminishes the risk of post-operative inflammation. This leads to better results (and lessened pain) for patients who require surgical implants.
The technology is also being used for glucose sensors and other biomedical monitors. These monitors use acrylic optical fibers that are textured by atomic oxygen. This texturing allows the fiber to filter out red blood cells, letting serum in the blood more effectively contact the chemical sensing component in the monitor.
'It makes the test more accurate… while requiring a fraction of the blood that's normally required to do blood sugar testing. You can prick almost anywhere on your body and get enough blood to register the sugar level of the blood,' says Sharon Miller, an electrical engineer in the Space Environment and Experiments Branch at NASA's Glenn Research Center.
Restoring Art
Damaged works of art can be restored and saved using atomic oxygen. This before and after image of 'Madonna of the Chair' shows the dramatic results that are possible. Photo Credit: NASAWhen works of art are compromised, atomic oxygen can be used to remove the organic contaminants without damaging the actual painting. The process removes all organic materials, such as carbon or soot, but it typically doesn't affect the paint. The pigments in paint are mostly inorganic, and have already been oxidized, meaning that atomic oxygen doesn't damage them. Pigments that are organic can also be preserved, through carefully timing the exposure to atomic oxygen. The canvas is also safe, as the atomic oxygen only reacts on the surface of the painting.
Artwork can be placed in a vacuum chamber where atomic oxygen is created. Depending on the amount of damage, the painting can remain in the chamber anywhere from 20 hours to 400 hours. The pencil beam can also be used to specifically target a damaged area in need of restoration, eliminating the need to place the artwork in a vacuum chamber.
Museums, galleries and churches have come to Glenn to save and restore their works of art. Glenn has demonstrated the ability to repair a fire-damaged painting by Jackson Pollack, removed lipstick from an Andy Warhol painting and saved smoke-damaged paintings at St. Stanislaus Church in Cleveland. The Glenn team has used atomic oxygen to restore a piece that was previously thought to be irreparable: a centuries-old, Italian copy of a painting by Raphael called 'Madonna of the Chair,' which belongs to St. Alban's Episcopal Church in Cleveland.
'[Atomic oxygen] is very effective. In art restoration, it works so well… it's not something you can buy in a bottle, but then again, it's more effective than what you can buy in a bottle,' Banks says.
Exploring the Future
The Atomic Oxygen Exposure Vacuum Chamber at Glenn enables cutting-edge research into the uses of atomic oxygen. Deborah Waters, of the Space Environment and Experiments Branch, works with the End Hall oxygen ion source. Photo Credit: NASANASA has worked, on a reimbursable basis, with a multitude of parties interested in atomic oxygen. Glenn has worked with individuals whose treasured artwork was damaged in home fires, and they have worked with corporations that are investigating atomic oxygen in biomedical applications, such as LightPointe Medical of Eden Prairie, Minn. They have discovered many applications for atomic oxygen, and look forward to investigating even more.
'There are a lot of avenues that haven't been fully explored,' Banks says. 'There have been a lot of space applications, but there are probably many more non-space applications.'
The team hopes to continue researching ways that atomic oxygen can be of use, and to further explore the promising areas that they have already identified. Many of the technologies have been patented, and the Glenn team hopes that companies will license and commercialize some of the technologies, so they may be of even more use to society.
'It would be nice to see more companies using technology derived from the nation's efforts in aerospace,' Banks says.
Oxygen Atom Ion
In certain conditions, atomic oxygen can cause damage. Thanks to NASA researchers, atomic oxygen is now used in ways that make positive contributions to space exploration and to life on Earth. Whether saving a priceless piece of art or enhancing human health, atomic oxygen is powerful.'It is very rewarding to work with because you see a benefit right away. It can have an immediate impact and benefit to the public,' Miller says.
-Tori Woods, SGT Inc.
NASA's Glenn Research Center
The milestone, which the MOXIE instrument achieved by converting carbon dioxide into oxygen, points the way to future human exploration of the Red Planet.
The growing list of “firsts” for Perseverance, NASA’s newest six-wheeled robot on the Martian surface, includes converting some of the Red Planet’s thin, carbon dioxide-rich atmosphere into oxygen. A toaster-size, experimental instrument aboard Perseverance called the Mars Oxygen In-Situ Resource Utilization Experiment (MOXIE) accomplished the task. The test took place April 20, the 60th Martian day, or sol, since the mission landed Feb. 18.
While the technology demonstration is just getting started, it could pave the way for science fiction to become science fact – isolating and storing oxygen on Mars to help power rockets that could lift astronauts off the planet’s surface. Such devices also might one day provide breathable air for astronauts themselves. MOXIE is an exploration technology investigation – as is the Mars Environmental Dynamics Analyzer (MEDA) weather station – and is sponsored by NASA’s Space Technology Mission Directorate (STMD) and Human Exploration and Operations Mission Directorate.
“This is a critical first step at converting carbon dioxide to oxygen on Mars,” said Jim Reuter, associate administrator STMD. “MOXIE has more work to do, but the results from this technology demonstration are full of promise as we move toward our goal of one day seeing humans on Mars. Oxygen isn’t just the stuff we breathe. Rocket propellant depends on oxygen, and future explorers will depend on producing propellant on Mars to make the trip home.”
For rockets or astronauts, oxygen is key, said MOXIE’s principal investigator, Michael Hecht of the Massachusetts Institute of Technology’s Haystack Observatory.
To burn its fuel, a rocket must have more oxygen by weight. To get four astronauts off the Martian surface on a future mission would require approximately 15,000 pounds (7 metric tons) of rocket fuel and 55,000 pounds (25 metric tons) of oxygen. In contrast, astronauts living and working on Mars would require far less oxygen to breathe. “The astronauts who spend a year on the surface will maybe use one metric ton between them,” Hecht said.
Hauling 25 metric tons of oxygen from Earth to Mars would be an arduous task. Transporting a one-ton oxygen converter – a larger, more powerful descendant of MOXIE that could produce those 25 tons – would be far more economical and practical.
Mars’ atmosphere is 96% carbon dioxide. MOXIE works by separating oxygen atoms from carbon dioxide molecules, which are made up of one carbon atom and two oxygen atoms. A waste product, carbon monoxide, is emitted into the Martian atmosphere.
The conversion process requires high levels of heat to reach a temperature of approximately 1,470 degrees Fahrenheit (800 Celsius). To accommodate this, the MOXIE unit is made with heat-tolerant materials. These include 3D-printed nickel alloy parts, which heat and cool the gases flowing through it, and a lightweight aerogel that helps hold in the heat. A thin gold coating on the outside of MOXIE reflects infrared heat, keeping it from radiating outward and potentially damaging other parts of Perseverance.
In this first operation, MOXIE’s oxygen production was quite modest – about 5 grams, equivalent to about 10 minutes’ worth of breathable oxygen for an astronaut. MOXIE is designed to generate up to 10 grams of oxygen per hour.
This technology demonstration was designed to ensure the instrument survived the launch from Earth, a nearly seven-month journey through deep space, and touchdown with Perseverance on Feb. 18. MOXIE is expected to extract oxygen at least nine more times over the course of a Martian year (nearly two years on Earth).
These oxygen-production runs will come in three phases. The first phase will check out and characterize the instrument’s function, while the second phase will run the instrument in varying atmospheric conditions, such as different times of day and seasons. In the third phase, Hecht said, “we’ll push the envelope” – trying new operating modes, or introducing “new wrinkles, such as a run where we compare operations at three or more different temperatures.”
“MOXIE isn’t just the first instrument to produce oxygen on another world,” said Trudy Kortes, director of technology demonstrations within STMD. It’s the first technology of its kind that will help future missions “live off the land,” using elements of another world’s environment, also known as in-situ resource utilization.
“It’s taking regolith, the substance you find on the ground, and putting it through a processing plant, making it into a large structure, or taking carbon dioxide – the bulk of the atmosphere – and converting it into oxygen,” she said. “This process allows us to convert these abundant materials into useable things: propellant, breathable air, or, combined with hydrogen, water.”
More About Perseverance
A key objective of Perseverance’s mission on Mars is astrobiology, including the search for signs of ancient microbial life. The rover will characterize the planet’s geology and past climate, pave the way for human exploration of the Red Planet, and be the first mission to collect and cache Martian rock and regolith (broken rock and dust).
Subsequent NASA missions, in cooperation with ESA (European Space Agency), would send spacecraft to Mars to collect these sealed samples from the surface and return them to Earth for in-depth analysis.
The Mars 2020 Perseverance mission is part of NASA’s Moon to Mars exploration approach, which includes Artemis missions to the Moon that will help prepare for human exploration of the Red Planet.
NASA’s Jet Propulsion Laboratory in Southern California, which is managed for NASA by Caltech in Pasadena, California, built and manages operations of the Perseverance rover.
For more about Perseverance:
and
Oxygen Atom Protons Neutrons Electrons
News Media Contacts
Karen Fox / Alana Johnson / Clare Skelly
Headquarters, Washington
301-286-6284 / 202-358-1501/ 202-515-6654
karen.c.fox@nasa.gov / alana.r.johnson@nasa.gov / clare.a.skelly@nasa.gov
Andrew Good
Jet Propulsion Laboratory, Pasadena, Calif.
818-393-2433
andrew.c.good@jpl.nasa.gov
Oxygen Atom Model
› more videos
